Quorum sensing, also sometimes referred to as autoinduction, is a process of cell–cell communication that enables organisms to detect and respond to their cell population density by regulating their gene expression. Although this phenomenon is most commonly observed in some bacteria, other organisms like some bacteriophages, some archaebacteria, and social insects like ants and honeybees. In addition to its function in biological systems, quorum sensing has several useful applications for computing and robotics. In general, quorum sensing can function as a decision-making process in any decentralized system in which the components have: a) a means of assessing the number of other components they interact with, and b) a standard response once a threshold number of components is detected. First reported in 1970 by Kenneth Nealson, Terry Platt, and J. Woodland Hastings with respect to bioluminescence in the marine bacterium Aliivibrio fischeri, it has been a hot topic of research in microbiology for the last two decades and largely popularised by a pioneer in this field, Bonnie Bassler.
Some of the best-known examples of quorum sensing come from studies of bacteria. Bacteria use quorum sensing to regulate certain phenotype expressions, which in turn, coordinate their behaviours. Some common phenotypes include biofilm formation, virulence factor expression, and motility. Certain bacteria are able to use quorum sensing to regulate bioluminescence, nitrogen fixation and sporulation. The quorum-sensing function is based on the local density of the bacterial population in the immediate environment. It can occur within a single bacterial species, as well as between diverse species. Both gram-positive and gram-negative bacteria use quorum sensing, but there are some major differences in their mechanisms (see picture below). For the bacteria to use quorum sensing constitutively, they must possess three characteristics: to secrete a signaling molecule, an autoinducer, to detect the change in concentration of signaling molecules, and to regulate gene transcription as a response. This process is highly dependent on the diffusion mechanism of the signaling molecules. Quorum sensing signaling molecules are usually secreted at a low level by individual bacteria. At low cell density, the molecules may just diffuse away. At high cell density, the local concentration of signaling molecules may exceed its threshold level, and trigger changes in gene expressions.

While the phenomenon of quorum sensing may seem really interesting, one might wonder the implications for humankind. This brings us to quorum quenching, the process of preventing quorum sensing by disrupting signalling. This is achieved by inactivating signalling enzymes, by introducing molecules that mimic signalling molecules and block their receptors, by degrading signalling molecules themselves, or by a modification of the quorum sensing signals due to an enzyme activity. Applications of quorum quenching that have been exploited by humans include the use of N-acylhomoserine lactone-degrading bacteria in aquacultures to limit the spread of diseases in aquatic populations of fish, mollusks and crustaceans. This technique has also been translated to agriculture, to restrict the spread of pathogenic bacteria that use quorum sensing in plants. Anti-biofouling is another process that exploits quorum quenching bacteria to mediate the dissociation of unwanted biofilms aggregating on wet surfaces, such as medical devices, transportation infrastructure and water systems. Quorum quenching is recently studied for the control of fouling and emerging contaminants in electro membrane bioreactors (eMBRs) for the advanced treatment of wastewater. Quorum sensing has been engineered using synthetic biological circuits in different systems. Examples include rewiring the AHL components to toxic genes to control population size in bacteria; and constructing an auxin-based system to control population density in mammalian cells. Synthetic quorum sensing circuits have been proposed to enable applications like controlling biofilms or enabling drug delivery. Quorum sensing can be a useful tool for improving the function of self-organizing networks such as the SECOAS (Self-Organizing Collegiate Sensor) environmental monitoring system. It can also be used to coordinate the behavior of autonomous robot swarms. Using a process similar to that used by Temnothorax ants, robots can make rapid group decisions without the direction of a controller. Some major areas of quorum sensing application are illustrated in the image below.
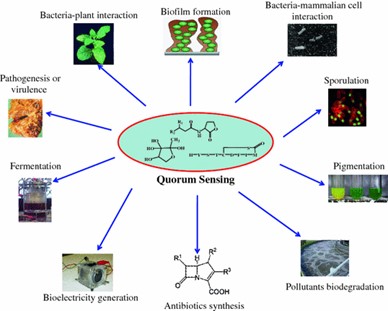
However, there are also some researchers raising issues with the basis of the development of quorum quenching therapy. For instance, despite the key role of QS signals in the virulence of many pathogens, the involvement of these signaling factors in the physiological processes of microorganisms is rarely taken into account. Also, one of the main assumptions of anti-QS therapy is the reduction of pathogens’ virulence by limiting the communication-dependent pathogenicity induction; there are, however, numerous scientific reports indicating that the dysfunction of genes responsible for the QS activity determines an increase of certain pathogenicity features. Another basic assumption of anti-QS therapies, apart from the selectivity and the reduction of microbial virulence, is the lack of the possibility for microorganisms to develop resistance mechanisms against this type of treatment. The challenges and limitations of quorum quenching therapy is summarized in the picture below.

In conclusion, there has been an explosion of activity on bacterial quorum sensing and the field continues to expand rapidly. One area ripe for advancement involves complex adaptive microbial communities. Quorum sensing likely controls behaviors critical for development and success of these communities in diverse environments like the human gut microbiome and chronic infections of humans. Elucidating the roles and functions of quorum sensing in natural ecosystems requires a continued willingness to embrace the complexity of these microbial communities and incorporate systems level ecological principles. We will continue to see incorporation of the fundamental biology of bacterial social dynamics into thinking about how to interfere with certain infectious diseases, and there will be a continued use of bacteria to understand the biology of communication and sociality. We have come to understand that there are many different small molecule-dependent interactions between microbes and between microbes and their hosts. There are certainly much more to be discovered, and there is an opportunity to sort out fundamental differences between these diverse systems. Understanding these issues will be critical as we move towards translating basic studies of quorum sensing to meet future needs including functional studies of the human microbiome. Hopefully, the researchers will very soon be able to address the challenges and move in the direction to fulfil the immense potential of this field.