Received: Wed 05, Aug 2020
Accepted: Wed 19, Aug 2020
Abstract
Aim: We investigated the expression of type-I gonadotropin-releasing hormone receptor (GnRHR-I) in human tongue cancer SCC-25 cells and the effects of gonadotropin-releasing hormone (GnRH)-I and its analog triptorelin on SCC-25 cells and the related mechanisms of action.
Methods: We used RT-PCR, real-time PCR (qPCR) and western blotting to detect the expression of GnRHR-I mRNA and protein in SCC-25 cells, We also used a Cell Counting Kit-8 (CCK-8) assay to calculate cell viability, after treatment with GnRH-I or triptorelin.
Results: Results of agarose gel electrophoresis and DNA sequencing showed that the GnRHR-I mRNA of SCC-25 cells were amplified correctly. Western blotting showed that the protein samples of SCC-25 cells had a specific reaction at approximately 60 kDa. GnRH or triptorelin inhibited the proliferation of SCC-25 cells in a dose-dependent manner. GnRH-I and triptorelin at the same concentrations did not significantly differ in inhibiting the proliferation of SCC-25 cells (10 µg/ml: P=0.44,20 µg/ml: P=0.57, 40µg/ml: P=0.28,80 µg/ml: P=0.051). Results of qPCR showed that triptorelin treatment led to stronger inhibition of GnRHR-I mRNA expression in SCC-25 cells than did GnRH-I treatment. (P<0.05). Triptorelin also decreased GnRHR mRNA expression time-dependently.
Conclusion: The SCC-25 cell line expresses GnRHR protein and mRNA. The inhibitory effect of GnRH-I and triptorelin on tongue cancer cells might be related to the downregulation of GnRHR-I mRNA.
1. Introduction
Gonadotropin-releasing hormone (GnRH) is a hypothalamic hormone that regulates reproduction [1]. The GnRH receptor (GnRHR) is expressed in both normal and cancerous human reproductive tissues (e.g., breast, endometrium, ovary, and prostate) [2]. However, the expression of GnRHR in tongue cancer cells or tissues has not been reported. We, therefore investigated whether tongue cancer SCC-25 cells express GnRHR. Studies have implied that GnRHR could have a novel role in tumor metastasis and angiogenesis [3]. Malignant neoplasms of the head and neck region account for 10% of all human malignant tumors; approximately 40% of head and neck tumors occur in the mouth [4]. Despite the combination of chemotherapy and radiotherapy, the prognosis is still poor for patients with tongue cancer; their 5-year survival rate is about 50% [5]. Tongue cancer has no specific biomarker, and available treatments are unaffordable for many people [6]. The tongue cancer survival rate has not greatly improved over recent years. We, therefore, need a targeting agent for tongue cancer with limited adverse effects. Whether GnRHR is expressed in tongue cancer cells is unclear. We selected a putative human tongue cancer cell line (SCC-25) as a research model and studied its GnRHR expression level, and how treatment with GnRH and GnRH analogs influence cell proliferation and GnRHR expression. Our findings could improve prognosis and provide the basis of new therapies.
2. Materials and Methods
2.1. Peptide Design and Synthesis
Two peptides were synthesized by China Peptides (Suzhou, China). Their amino acid sequences were GnRH-I: pGlu-His-Trp-Ser-Tyr-Gly-Leu-Arg-Pro-Gly-NH2; and Triptorelin: pGlu-His-Trp-Ser-Tyr-Trp-Leu-Arg-Pro-Gly-NH2. The peptides were purified by preparative reversed-phase HPLC (GnRH-I: 96.63%; triptorelin: 97.64%) and had the expected amino acid compositions and mass spectra.
2.2. Cell Culture
The cell line SCC-25 was presented by Professor Sun Hongchen, School of Stomatology, Jilin University. We used HeLa cells (human cervical carcinoma) as positive controls and Jurkat cells (human T lymphocyte cells) as negative controls; they were obtained from the Academy of Military Medical Sciences. We maintained the SCC-25 cells in DMEM medium (GIBCO, California, USA), and the HeLa and Jurkat cells in RPMI-1640 medium (GIBCO) supplemented with 10% fetal bovine serum (GIBCO) in a humidified incubator with a mixture of 5% CO2 at 37°C.
2.3. RT-PCR and Real-Time PCR
Total RNA from the SCC-25, HeLa and Jurkat cells was extracted using Super Total RNA Extraction Kit (Promega, Wisconsin, USA) and cDNA was synthesized from 1 µg of total RNA according to the manufacturer’s protocol (Takara, Beijing, China). Primer sequences for PCR were GnRHR: 5′-GCTCTCTGCGACCTTTA-3′ (forward) and 5′-TGTTCCACATCCCATCC-3′ (reverse); Beta-2 microglobulin (β2-M): 5′-GGGTTTCATCCATCCGACATT-3′ (forward) and 5′-GGCATGGACTGTGGTCATGAG-3′ (reverse). The PCR procedures were: incubation at 94°C for 5 min; 40 cycles at 94°C for 30 s, 60°C for 30s and 72°C for 40s; ending with a final extension for 5 min at 72°C. To verify the accuracy of the amplification, the RT-PCR products were electrophoresed through a 1.5% agarose gel stained with ethidium bromide; the gel was then collected to be sequenced by Jilin Comate Bioscience Co., Ltd.
Quantitative real-time PCR (qPCR) was carried out on a 7500 QPCR System (Applied Biosystems, California, USA) to detect the GnRHR-I mRNA level of SCC-25 cells treated with 40 µg/ml GnRH-I or triptorelin for 48 hours, or treated with triptorelin for 0, 12, 24, 36 or 48 hours. The Ct value was defined as the cycle number at which the fluorescence intensity reached a certain threshold where the amplification of each target gene was within the linear region of the reaction amplification curves. Gene expression was normalized to β2-M expression. Expression changes were calculated using the ΔΔCt method.
2.4. Western Blot Analysis
Proteins were extracted from the three cell lines with RIPA buffer (Beyotime Institute of Biotechnology, Suzhou, China) in the presence of the protease inhibitor phenylmethylsulfonyl fluoride (Amresco, USA). Following quantification of the total proteins, the proteins were separated by 12% polyacrylamide gel electrophoresis and transferred to polyvinylidene difluoride membranes (Millipore, USA). The membranes were blocked with 5% dried skim milk in phosphate-buffered saline-Tween 20. After incubation with a monoclonal mouse anti-human GnRHR antibody (diluted 1:1000, ab24095, Abcam, USA) overnight at 4°C, membranes were incubated with a secondary horseradish peroxidase-conjugated goat anti-mouse antibody (1:2000, ZSGB-Bio, China) for 2 h. Blots were visualized by chemiluminescence (FluorChem HD2, Alpha Innotech, USA) and analysed with Image J.
2.5. Cell Viability and Proliferation
To investigate the effects of GnRH-I and triptorelin on cell viability, SCC-25 cells (8×103/well) were seeded into 96-well plate and pre-incubated for 12 hours in a humidified incubator (37°C, 5% CO2). Cells were randomly divided into two groups, with 5 repeated wells used for each group. For the GnRH-I group and triptorelin group, GnRH-I and triptorelin at different concentrations (0, 10, 20, 40 or 80 µg/ml) were added to the SCC-25 cells. We set 0 µg/ml group as the control group. After treatment for 48 hours, we added 10 µl of CCK-8 (Dojindo, Japan) solution to each well. We incubated the plate for 90 minutes in an incubator and then measured the absorbance at 450 nm using a microplate reader (IF500, Tecan, Switzerland). Viability of cells treated with no drugs was set at 100%, and absorbance of wells with the medium but no cells was set to 0%.
2.6. Statistical Analysis
Data are expressed as mean ± standard error. Statistical analysis was performed using Student’s t test and one-way ANOVA on SPSS software, version 22.0 (SPSS, Inc., Chicago, IL, USA). P<0.05 was considered significant.
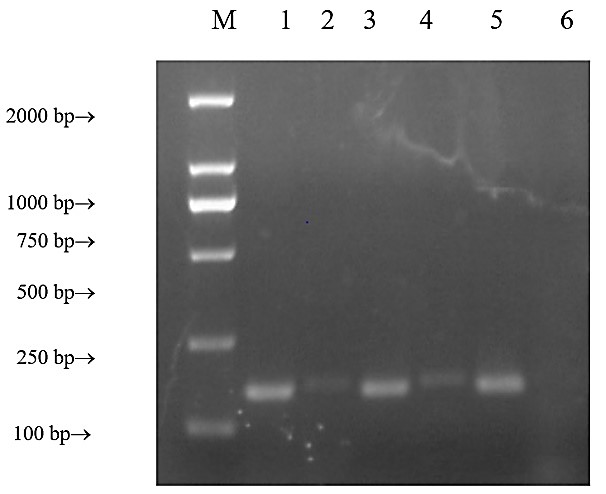
3. Results
3.1. GnRHR mRNA and Protein Expression in SCC-25 Cell Line
Our PCR results showed bands in the reference gene and the HeLa GnRHR-I lanes (positive control), but no bands in the Jurkat GnRHR-I lane negative control); positioning of PCR products of the reference gene was correct. The SCC-25 GnRHR-I lane formed a single band at 167 bp, and the primers had good specificity (Figure 1). The gel-cutting recovery sequencing was verified by homologous alignment in the NCBI Blast database, which confirmed the existence of GnRHR-I gene expression in SCC-25 cells.
Western blotting showed specific reaction bands at 60 kDa for SCC-25 cell protein; a band could also be observed at 60 kDa for HeLa cells and indicated GnRHR-I expression, but no such band was seen for the Jurkat cells. The 3 reference proteins showed good response to antibody. No bands were found for SCC-25 proteins that had only incubated with the second antibody, which confirmed the specificity of the second antibody. The results showed GnRHR-I protein expression in SCC-25 cells. Gray-scale scanning analysis with ImageJ software showed that the expression of the HeLa cell protein was 1.67 ± 0.19 times that of SCC-25 cells. The results showed that the expression of GnRHR-I protein in SCC-25 cells was different from that in HeLa cells (P=0.016) (Figure 2).
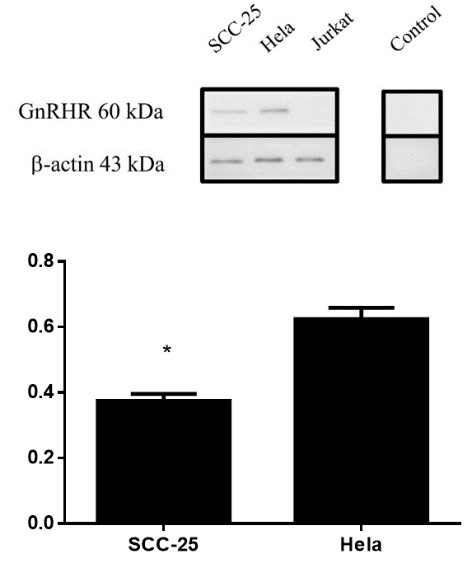
3.2. Effect of GnRH and Triptorelin on Proliferation of SCC-25 Cells In Vitro
Single-factor variance analysis showed no significant difference in the viability of SCC-25 cells treated with 10 µg/ml GnRH-I for 48 hours compared with the control group (0 µg/ml; P=0.171). The survival rate of SCC-25 cells treated with 20 µg/ml GnRH-I for 48 hours was significantly different from that of the control group (P=0.018). The survival rate of SCC-25 cells treated with 40 and 80 µg/ml GnRH-I were significantly different from those of the control group (P=0.002, and P=0.0001, respectively).
The survival rates of SCC-25 cells treated with 10 µg/ml triptorelin for 48 hours, and the control group (0 µg/ml) did not significantly differ (P=0.118). But it did significantly differ when SCC-25 cells were treated with 20 µg/ml triptorelin for 48 hours (P=0.02). Survival rates of SCC-25 cells treated with 40 and 80 µg/ml triptorelin were also significantly different from those of the control group (P=0.002, and P=0.00001, respectively). Cell viability SCC-25 did not significantly differ between cells treated with similar concentrations of GnRH-I and triptorelin (Figure 3).
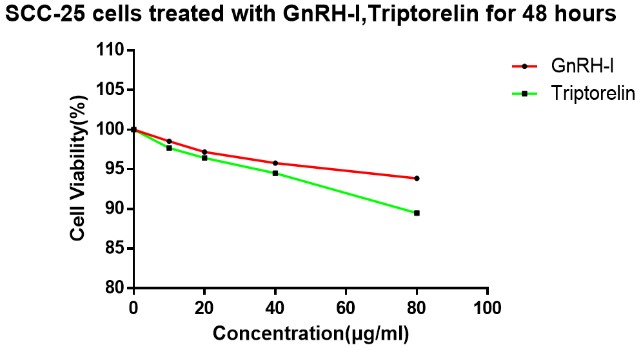

We analysed qPCR results by independent t-test. GnRHR-I mRNA levels of SCC-25 cells significantly decreased after treatment with 40 µg/ml GnRH-I or 40 µg/ml triptorelin for 48 hours. Compared with the control group (0 µg/ml, 48 hours), expression of GnRHR-I mRNA was downregulated in cells treated with GnRH-I (0.256±0.023 × control group, P=0.001) and with triptorelin (0.117±0.013 × control group, P=0.001). The difference in mRNA expression between cells treated with 40µg/ml GnRH-I and those treated with 40 µg/ml triptorelin was extremely significant (P=0.0008); triptorelin showed a stronger inhibitory effect on SCC-25 GnRHR-I mRNA expression levels. GnRHR-I mRNA expression levels in SCC-25 cells were significantly downregulated after treatment with 40 µg/ml triptorelin for 24, 36, and 48 hours but not at 12 hours compared with the control group (0 hours). The mRNA expression level of the 24-hour/40 µg/ml triptorelin treatment group was 0.565±0.016× that of the control group(0 µg/ml,48 hours), 0.250±0.02× that of the control group in the 36-hour treatment group, and 0.175±0.034× that of the control group in the 48-hour treatment group (12-hour group: t=1.983, P=0.186; 24-hour group: t=25.734, P=0.002; 36-hour group: t=19.397, P=0.003; 48-hour group: t = 107.60, P < 0.0001) (Figures 4 & 5).
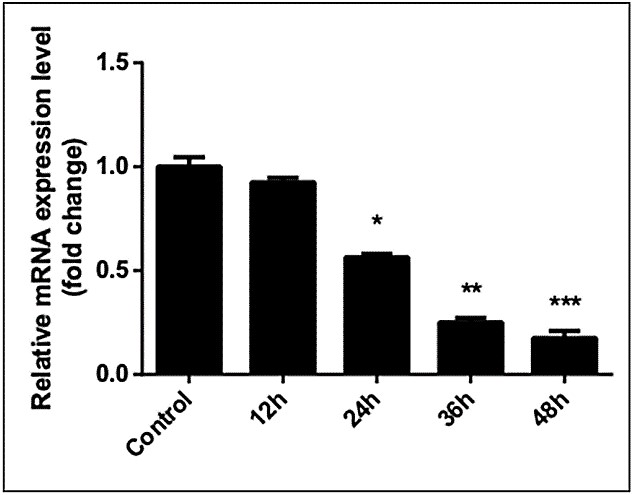
4. Discussion
GnRH is the pivotal hormone in the hypothalamic-pituitary-gonadal axis, and GnRHR are trans-membrane G-protein-coupled receptors [7, 8]. Two types of GnRH exist in mammals, and GnRH-I was first discovered by Guillemin in 1967 [9]. Its specific binding to GnRHR-I plays an important role in the reproductive system, and its role in regulating the pituitary secretion of gonadotropins is well known. This hypothalamic-pituitary-gonadal axis that regulates fertility is found in humans and many other vertebrates, such as fish, birds and reptiles [10-12].
According to studies by G. Lee et al., many normal or cancerous cell lines from different sources express GnRHR-I, including breast cancer cell lines MCF7, MDA-MB-231, MDA-435 and T-47D; cervical cancer cell lines C-33A, SiHa and ME-180; colon cancer cell lines HCT 115, HCT 116, HT-29 and SW-48; glioblastoma cell line HTB-14; liver cancer cell lines Hep3B and HepG2; laryngeal cancer cell line Hep-2; lung cancer cell lines A549, Calu-6 and H441; lymphoma cell line HEL 1; melanoma cell lines MMAN, MMRU and SK-MEL-3; neuroblastoma SH-SY5Y, ovarian cancer cell line SK-OV-3,OC-3-VGH and OVCAR-3; human choriocarcinoma cell line JEG3, and prostate cancer cells DU 145 and PC-3 [13]. Here we have confirmed for the first time that GnRHR is also expressed in SCC-25 cells, and GnRHR is widely present on the surface of cancer cells.
A GnRH agonist could be a targeted drug. Currently, triptorelin, goserelin and leuprolide are used in the clinical treatment of prostate cancer, breast cancer, uterine fibroids, endometriosis and various sex hormone-related diseases. The amino acid sequence of triptorelin is replaced by tryptophan in place of glycine in the native sequence, compared with type I gonadotropin-releasing hormone. In humans, the half-life of GnRH-I is only 2-5 minutes before cleavage by serum proteases; GnRH agonists, including triptorelin, can extend its half-life and increase binding to receptors [14]. In the treatment of prostate cancer, triptorelin is a first-line hormone therapy and can be efficacious and safe for patients with advanced prostate cancer [15].
The expression of GnRH and its receptor is a part of an autocrine regulatory system of cell proliferation that has been demonstrated in several human malignancies. Many researchers have observed dose-dependent antiproliferative effects of GnRH agonists in cell lines derived from these cancers [16]. Our results can confirm that 40 µg/ml GnRH-I and triptorelin can significantly inhibit the proliferation of SCC-25 cells after 48 hours of treatment, and downregulate mRNA of GnRHR-I in SCC-25 cells. The expression of GnRHR mRNA in the 40 µg/ml triptorelin group was lower than that in the 40 µg/ml GnRH group (P<0.01). Therefore, in subsequent experiments, the treatment of SCC-25 cells with 40 µg/ml triptorelin for 0–48 hours showed time-dependent down-regulation of GnRHR mRNA.
These results indicate that GnRH-I-mediated changes in GnRHR-I mRNA in SCC-25 cells and the simultaneous inhibition of tumor cell proliferation are not unique to reproductive system tumors; triptorelin also inhibits proliferation of SCC-25 cells and downregulates GnRHR mRNA. In the nervous system, GnRH-I stimulates the pituitary gland to produce gonadotropin in a pulsed release. This pulsed release helps maintain the level of the pituitary GnRHR-I and avoids its downregulation through continuous stimulation [17]. The most important features of GnRH signaling in tumors are the inhibition of the mitogenic pathway, which suppresses proliferation, and may protect cells from apoptosis via activation of NFκB [16]. The effects of GnRH and its receptors in tongue cancer cells need further study.
In conclusion, SCC-25 cells express GnRHR protein and mRNA. The inhibitory effect of GnRH-I and triptorelin on SCC-25 cells might reflect downregulated GnRHR-I mRNA.
Acknowledgements
This study was supported by the Natural Science Foundation of Jilin Province (Grant No. 20160101095JC).
Conflicts of Interest
None.
REFERENCES
- Robert P Millar “GnRHs and GnRH receptors.” Anim Reprod Sci, vol. 88, no. 1-2, pp. 5, 2005. View at: Publisher Site | PubMed
- Patrizia Limonta, Roberta M Moretti, Marina Montagnani Marelli, et al. “The biology of gonadotropin hormone-releasing hormone: role in the control of tumor growth and progression in humans.” Front Neuroendocrinol, vol. 24, no. 4, pp. 279-295, 2003. View at: Publisher Site | PubMed
- Cheung LW, Wong AS “Gonadotropin-releasing hormone: GnRH receptor signaling in extrapituitary tissues.” FEBS J, vol. 275, pp. 5479-5495, 2008. View at: Publisher Site | PubMed
- Renato Paladino Nemoto, Alana Asciutti Victorino, Gregory Bittar Pessoa, et al. “Oral cancer preventive campaigns: are we reaching the real target?” Braz J Otorhinolaryngol, vol. 81, no. 1, pp. 44-49, 2015. View at: Publisher Site | PubMed
- “Global oral cancer incidence.” Br Dental J, vol. 221, no. 6, pp. 288, 2016. View at: Publisher Site
- César Rivera “Essentials of oral cancer.” Int J Clinical Exp Pathol, vol. 8, no. 9, pp. 11884-11894, 2015. View at: PubMed
- Zvi Naor, Ilpo Huhtaniemi “Interactions of the GnRH receptor with heterotrimeric G proteins.” Front Neuroendocrinol, vol. 34, no. 2, pp. 88-94, 2013. View at: Publisher Site | PubMed
- Hanna Pincas, Soon Gang Choi, Qian Wang, et al. “Outside the box signaling: secreted factors modulate GnRH receptor-mediated gonadotropin regulation.” Mol Cell Endocrinol, vol. 385, no. 1-2, pp. 56-61, 2014. View at: Publisher Site | PubMed
- R Guillemin “The adenohypophysis and its hypothalamic control.” Ann Rev Physiol, vol. 29, pp. 313-348, 1967. View at: Publisher Site | PubMed
- A Y Al Kindi, Y Mahmoud, M J Woller “Ultrastructural changes in granulosa cells and plasma steroid levels after administration of luteinizing hormone-releasing hormone in the Western painted turtle, Chrysemys picta.” Tissue Cell, vol. 33, no. 4, pp. 361-367, 2001. View at: Publisher Site | PubMed
- R C Francis, K Soma, R D Fernald “Social regulation of the brain-pituitary-gonadal axis [J].” Proc Natl Acad Sci U S A, vol. 90, no. 16, pp. 7794-7798, 1993. View at: Publisher Site
- V B Mahesh, T G Muldoon, E J Smanik, et al. “Interaction between the ovaries and the hypothalamic pituitary axis in the regulation of gonadotropin secretion.” Prog Clin Biol Res, vol. 112, no. A, pp. 165-182, 1982. View at: PubMed
- Gregory Lee, Bixia Ge “Growth inhibition of tumor cells in vitro by using monoclonal antibodies against gonadotropin-releasing hormone receptor.” Cancer Immunol Immunother, vol. 59, no. 7, pp. 1011-1019, 2010. View at: Publisher Site | PubMed
- Dorothea Weckermann, Rolf Harzmann “Hormone Therapy in Prostate Cancer: LHRH Antagonists versus LHRH Analogues.” Eur Urol, vol. 46, no. 3, pp. 279-284, 2004. View at: Publisher Site | PubMed
- Axel S Merseburger, Marie C Hupe “An Update on Triptorelin: Current Thinking on Androgen Deprivation Therapy for Prostate Cancer.” Adv Ther, vol. 33, no. 7, pp. 1072-1093, 2016. View at: Publisher Site | PubMed
- Carsten Gründker, Andreas R Günthert, Silke Westphale, et al. “Biology of the gonadotropin-releasing hormone system in gynecological cancers.” Eur J Endocrinol, vol. 146, no. 1, pp. 1-14, 2002. View at: Publisher Site | PubMed
- Liu F, Austin D A, Webster N J “Gonadotropin-releasing hormone-desensitized LbetaT2 gonadotrope cells are refractory to acute protein kinase C, cyclic AMP, and calcium-dependent signaling.” Endocrinology, vol. 144, no. 10, pp. 4354-4365, 2003. View at: Publisher Site